Draft:Original research/Classical mechanics
In physics relating to or based upon concepts and theories that preceded the theories of relativity and quantum mechanics; such as Newtonian physics are called classical. Usually, in other fields, classical refers to the classical history period which dates from around 2,000 to 1,000 b2k.
Introduction
[edit | edit source]Classical mechanics is the study of everyday forces which we come in contact with. The goal of classical physics is to be able to describe perfectly any and all kinds of motion. From this it would follow that if you knew only part of the motion of an object, then you could determine each other part of the motion.
Theoretical classical mechanics
[edit | edit source]Def. an "[amount of] intervening space between two points,[1] usually geographical points, usually (but not necessarily) measured along a straight line"[2] is called a distance.
Def. the "inevitable progression into the future with the passing of present events into the past"[3] or the "inevitable passing of events from future to present then past"[4] is called time.
Def. the "quantity of matter which a body contains, irrespective of its bulk or volume"[5] or a "quantity of matter cohering together so as to make one body, or an aggregation of particles or things which collectively make one body or quantity"[6] is called a mass.
Def. the "rate of motion or action, specifically[7] the magnitude of the velocity;[8] the rate distance is traversed in a given time"[9] is called the speed.
Positions
[edit | edit source]position | m |
angular position/angle | unitless (radian) |
velocity | m·s−1 |
angular velocity | s−1 |
acceleration | m·s−2 |
angular acceleration | s−2 |
jerk | m·s−3 |
"angular jerk" | s−3 |
specific energy | m2·s−2 |
absorbed dose rate | m2·s−3 |
moment of inertia | kg·m2 |
momentum | kg·m·s−1 |
angular momentum | kg·m2·s−1 |
force | kg·m·s−2 |
torque | kg·m2·s−2 |
energy | kg·m2·s−2 |
power | kg·m2·s−3 |
pressure and energy density | kg·m−1·s−2 |
surface tension | kg·s−2 |
spring constant | kg·s−2 |
irradiance and energy flux | kg·s−3 |
kinematic viscosity | m2·s−1 |
dynamic viscosity | kg·m−1·s−1 |
density (mass density) | kg·m−3 |
density (weight density) | kg·m−2·s−2 |
number density | m−3 |
action | kg·m2·s−1 |
In pre-Einstein relativity (known as Galilean relativity), time is considered an absolute, i.e., the time interval that is observed to elapse between any given pair of events is the same for all observers.[10]
In addition to relying on absolute time, classical mechanics assumes Euclidean geometry for the structure of space.[11]
Motions
[edit | edit source]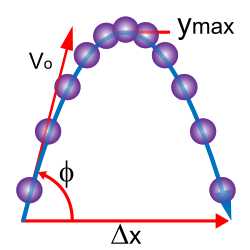
Essentially, motion boils down to where an object is, how fast it is going, and how fast it is changing how fast it is going.
In strict physical terms, these quantities are:
Position: the location of an object (generally from its centre of gravity) in relation to all other objects in a system.
Velocity: the rate at which the position changes.
Acceleration: The rate at which the object's velocity changes.
The units used to describe these quantities are:
- meter (m): the standard unit for distance in one direction. A meter is about 39 inches, or slightly more the three feet.
- second (s): the standard unit for time. a second is the time that elapses during 9,192,631,770 vibrations of the cesium 133 atom.
These relate to each other as follows:
Position = x (in meters)
Speeds and velocity
[edit | edit source]Velocity = change in position (distance d) divided by change in time (t)
- .
The velocity of the first object as seen by the second object is
Similarly, the first object sees the velocity of the second object as
When both objects are moving in the same direction, this equation can be simplified to
Or, by ignoring direction, the difference can be given in terms of speed only:
Accelerations
[edit | edit source]Acceleration = change in velocity (v) divided by change in time
- a = (change in v)/t
for example, if I were to walk in a straight line 10 m, and it took me 5 s to do this, my velocity would be:
- v = (change in d)/t
- v = 10 m/5 s
- v = 2 m/s
my velocity was 2 m/s
In a similar fashion, if you knew my initial velocity to be 2 m/s and after a 20 s stretch, my velocity was 42 m/s, my acceleration would be
- a = (change in v)/t
- a = (42 m/s - 2 m/s)/20 s
- a = (40 m/s)/20 s
- a = 2 m/s²
my acceleration was 2 m/s²
Reference frames
[edit | edit source]Consider two reference frames S and S'. For observers in each of the reference frames an event has space-time coordinates of (x,y,z,t) in frame S and (x',y',z',t') in frame S'. Assuming time is measured the same in all reference frames, and if we require x = x' when t = 0, then the relation between the space-time coordinates of the same event observed from the reference frames S' and S, which are moving at a relative velocity of u in the x direction is:
Spectra of motions
[edit | edit source]In physics, there is a distinction between average velocity and instantaneous velocity. Instantaneous velocity is the velocity at a specific point in time, and can be only derived with advanced calculus which may be covered in an advanced section. Average velocity, however, is what we will be dealing with. Average velocity involves the difference between two points in space and the difference in time associated with them. This is different from average speed. Average speed is the distance traveled divided by the time expended. Speed is based on the path an object takes, while velocity is based on the displacement of an object, i.e. the amount of distance it actually covered. for example, if I were to move 10 m to the left, and then 10 m to the right, over the course of 1 s, my path would be 20 m long, but my displacement would be 0 m, because the starting point and ending point were the same. Therefore, my average speed would be 20 m/s, while my velocity would be 0 m/s. The difference is important, because in physics, speed is never used, only velocity. The reason for this is that a physicist is considered an outside observer, and should be able to describe the event perfectly without actually witnessing what occurred. If only two data points are taken, then velocity is the only way to calculate things because no data has been taken in the middle, and no data about the path can be known.
Masses
[edit | edit source]Long ago, in the 1600's, a man named Isaac Newton established three laws relating to motion, which brought physics out of what was described above to a new level.
His first law was that each object will stay in motion unless acted upon by an unbalanced outside force, and likewise for an object at rest. This established a thing called momentum, which is a property of matter based upon an object's mass and velocity. An object, like a train moving at 10 m/s, will have more momentum than, say, a pea at the same velocity. This means that, in the same amount of time, the train will require more energy to stop than the pea. However, velocity also plays a role, as a 20 m/s train will be harder to stop than a 10 m/s train.
Forces
[edit | edit source]His second law stated that force equals mass multiplied by acceleration. Derivation of F = ma from Newton's original definition (Force is the rate of change of momentum)
Then, if mass is kept constant, , and .
Newton's Laws
[edit | edit source]Classical Mechanics is the study of the motion of particles and systems of particles and of the forces which induce changes in motion. Quantum mechanics and Relativity, developed in the 20th century, were advancements, which corrected classical mechanical errors in motion. However, since classical mechanics is easier to understand and widely applicable to most everyday physical situations it is still quite useful today.
Classical Mechanics were described in detail by Sir Isaac Newton in his two volume Philosophiae Naturalis Principia Mathematica in the 17th century, sometimes referred to as Principia or Principia Mathematica. We will begin our study of classical mechanics with Newton's three laws of motion.
1) An object at rest will remain at rest unless acted upon by an external force. An object in motion will move at a constant velocity unless acted upon by an external force.
This first law was a major advancement in physics, as it was previously assumed that an object in motion on earth would always return to a state of rest unless acted on by a force. We now understand that the force of friction must act upon the object to return it to rest.
2) Force = the change in momentum of a particle (or system of particles) with respect to time.
This law is often portrayed in the simple equation,
which is true when the mass is constant. A derivation of this simpler equation is displayed below.
3) Every action has an equal and opposite action.
This can be displayed by pushing on the wall or firing a gun. When I apply a force to the wall, the wall in turn applies an equal and opposite force on me. When the propellant in a gun is ignited the resulting chamber pressure propels the bullet forward and an equal force pushes the gun backward, providing the kickback force.
See also
[edit | edit source]- Agriculture (Middle Bronze Age) (15 kB) (13 September 2019)
- Agronomy (3 kB) (24 July 2018)
- Archaeology (-12th Century) (70 kB) (10 September 2019)
- Astrohistory (118 kB) (19 October 2019)
- Cenozoic (Danian) (231 kB)
- Classical mechanics (11 kB) (4 January 2020)
- Classical planets (Hasselo stadial) (109 kB)
- Comets (36 kB) (6 December 2019)
- Conditions (8 kB) (23 October 2019)
- Control groups (41 kB) (24 November 2019)
- Dates (Hadean) (173 kB) (31 August 2019)
- Draft:Distances (12 kB) (6 October 2019)
- Gases (23 kB) (27 October 2019)
- Geochronology (124 kB) (13 August 2019)
- Geographic coordinates (37 kB) (12 August 2019)
- Geophysics (80 kB) (12 August 2019)
- Geoseismology (36 kB) (7 December 2019)
- History (Hadean) (163 kB) (3 August 2019)
- How-to's (3 kB) (18 December 2019)
- Ice cores (Calabrian) (100 kB) (29 March 2019)
- Draft:Original research/Interstellar vehicles (15 kB) (9 November 2019)
- Lofting technology (91 kB) (22 December 2019)
- Measurements (3 kB) (12 December 2019)
- Mining geology (24 kB) (16 August 2019)
- Particle fountain (11 kB) (6 November 2019)
- Petrophysics (40 kB) (7 November 2019)
- Physics (30 kB) (8 December 2019)
- Proof of technology (13 kB) (30 August 2019)
- Safety (13 kB) (13 December 2019)
- Sciences (-55th Century) (101 kB) (24 September 2019)
- Sedimentary rocks (48 kB) (31 August 2019)
- Sediments (57 kB) (19 September 2019)
- Shielding (10 kB) (19 August 2019)
- Spaceflights (23 kB) (11 December 2019)
- Technology (48 kB) (31 December 2019)
References
[edit | edit source]- ↑ Emperorbma (17 August 2003). "distance". San Francisco, California: Wikimedia Foundation, Inc. Retrieved 24 May 2019.
{{cite web}}
:|author=
has generic name (help) - ↑ Brya (17 January 2006). "distance". San Francisco, California: Wikimedia Foundation, Inc. Retrieved 24 May 2019.
{{cite web}}
:|author=
has generic name (help) - ↑ DAVilla (3 January 2009). "time". San Francisco, California: Wikimedia Foundation, Inc. Retrieved 23 July 2019.
{{cite web}}
:|author=
has generic name (help) - ↑ 24.13.132.38 (23 September 2005). "time". San Francisco, California: Wikimedia Foundation, Inc. Retrieved 23 July 2019.
{{cite web}}
:|author=
has generic name (help) - ↑ Eclecticology (12 September 2003). "mass". San Francisco, California: Wikimedia Foundation, Inc. Retrieved 2013-08-12.
{{cite web}}
:|author=
has generic name (help) - ↑ Emperorbma (14 November 2003). "mass". San Francisco, California: Wikimedia Foundation, Inc. Retrieved 2014-02-28.
{{cite web}}
:|author=
has generic name (help) - ↑ Widsith (15 May 2006). "speed". San Francisco, California: Wikimedia Foundation, Inc. Retrieved 4 January 2020.
{{cite web}}
:|author=
has generic name (help) - ↑ Connel MacKenzie (23 November 2005). "speed". San Francisco, California: Wikimedia Foundation, Inc. Retrieved 4 January 2020.
{{cite web}}
:|author=
has generic name (help) - ↑ Emperorbma (14 November 2003). "speed". San Francisco, California: Wikimedia Foundation, Inc. Retrieved 4 January 2020.
{{cite web}}
:|author=
has generic name (help) - ↑ Knudsen, Jens M.; Hjorth, Poul (2012). Elements of Newtonian Mechanics (illustrated ed.). Springer Science & Business Media. p. 30. ISBN 978-3-642-97599-8. https://books.google.com/books?id=rkP1CAAAQBAJ&pg=PA30.
- ↑ "Physics lecture" (PDF). 2013-07-09.
External links
[edit | edit source]
Learn more about Classical mechanics |